We solve the most challenging issues ..!!

The increasing worldwide trend towards environmentally friendly and sustainable energy solutions has generated considerable attention towards the creation of green hydrogen. Green hydrogen, often known as the most sought-after solution for sustainable energy, is generated by the process of electrolysis, wherein water is split into hydrogen and oxygen, using renewable energy sources like wind and solar power. Electrochemical processes are of paramount importance in this undertaking, with several electrolyzer methodologies taking the forefront. This essay explores the complex realm of green hydrogen production, focusing on the difficulties encountered by technology providers and the advancements revolutionizing the domain of electrochemical processes and electrolyzer technologies, encompassing Alkaline (ALK), Proton Exchange Membrane (PEM), Solid Oxide Electrolysis Cell (SOEC), and Anion Exchange Membrane (AEM).
The present topic revolves on the challenges encountered in the production of green hydrogen.
The generation of green hydrogen is strongly dependent on intermittent renewable energy sources such as wind and solar, which exhibit sporadic patterns of availability. The primary objective is the development of highly effective energy storage systems in order to guarantee continuous electrolysis processes.
The efficiency of electrolyzers is crucial in order to minimize energy consumption and associated expenses. The attainment of a high conversion efficiency continues to pose a substantial obstacle.
The durability of electrolyzers is a critical factor since the components must be capable of enduring challenging circumstances, such as elevated temperatures and corrosive surroundings. The maintenance of long-term durability is of utmost importance in order to guarantee the economic sustainability of hydrogen generation.
The purity requirements for green hydrogen are quite rigorous, particularly in the context of industrial and transportation uses. Ensuring and maintaining elevated levels of purity throughout the manufacturing process is a significant challenge.
The process of expanding the production of green hydrogen to satisfy increasing demand requires significant investments in infrastructure and technology, hence posing noteworthy technical and economic obstacles.
The study examines the electrochemical processes involved in the production of green hydrogen.
Electrochemical techniques serve as the fundamental basis for the creation of green hydrogen. The process involves the use of electrolysis to separate water molecules into hydrogen and oxygen gases. The essential components of an electrolysis system include the electrolyzer stack, electrodes, and the electrolyte.
Alkaline electrolysis (ALK) is a process that operates on the principle of using a liquid alkaline electrolyte, often potassium hydroxide (KOH), to enable the exchange of ions.
One of the primary challenges associated with ALK electrolyzers is their historical tendency to exhibit larger physical dimensions and worse overall efficiency compared to other technologies. The task of surmounting these constraints is a formidable challenge.
The advancements in ALK technology primarily aim to boost efficiency, minimize system dimensions, and integrate PEM parts to optimize performance.
Proton Exchange Membrane Electrolysis (PEM): The operational principle of PEM electrolysis involves the use of a solid membrane that conducts protons as the electrolyte. This design feature serves to improve overall efficiency and minimize the occurrence of gas crossover.
One of the challenges associated with PEM electrolysis is the need for high quality water, which renders water treatment and filtering a crucial obstacle. The durability of catalysts is also a matter of concern.
The research endeavors in the field of innovations primarily concentrate on the development of highly sophisticated catalyst materials and durable membranes with the aim of augmenting both efficiency and lifespan.
The Solid Oxide Electrolysis Cell (SOEC) functions by using elevated temperatures and a solid ceramic electrolyte to facilitate the decomposition of water vapor into hydrogen and oxygen.
One of the primary challenges in this field is to the attainment of high-temperature operation, while simultaneously ensuring durability and efficiency. The selection of materials and the control of heat conditions are critical concerns.
The progress in materials research, particularly the emergence of novel ceramic materials, is facilitating advancements in efficiency.
Anion Exchange Membrane Electrolysis (AEM) operates on a similar principle to Proton Exchange Membrane (PEM) electrolysis, with the key difference being the use of an anion-conductive membrane.
There are many issues associated with anion exchange membranes (AEMs) in the field of electrochemical energy conversion. Two prominent challenges are ensuring stability and endurance of AEMs, as well as the creation of effective anion exchange materials.
The research endeavors in the field of innovations mostly concentrate on augmenting the stability of anion exchange membranes (AEMs) and identifying appropriate materials for anion exchange.
The use of advanced catalysts, such as platinum group metals (PGMs) and non-PGM catalysts, has led to notable improvements in electrolyzer efficiency and cost reduction.
Nanotechnology has emerged as a promising field for enhancing the efficacy of electrodes and membranes within electrolysis systems via the use of nanomaterials.
The field of hydrogen storage is currently seeing advancements in technology aimed at overcoming obstacles associated with the transport and storage of hydrogen. These innovations include solid-state hydrogen storage and liquid organic hydrogen carriers (LOHCs).
The use of smart control systems and automation in the field of automation and control systems is enhancing the operational performance of electrolyzers and augmenting overall system efficiency.
The maintenance of high hydrogen purity throughout manufacturing is being facilitated by the use of advanced sensors and monitoring systems.
The integration of hydrogen generation with other industrial processes, such as ammonia production or steelmaking, is leading to advancements in the overall sustainability of green hydrogen.
The applications and potential impact of a given subject or technology are crucial considerations in academic discourse. Understanding the practical uses and potential consequences of a concept or innovation is essential for evaluating its significance and relevance. By examining the Green hydrogen has the capacity to effectively tackle worldwide energy and environmental issues, presenting significant opportunities.
The process of decarbonization may be significantly facilitated by the use of hydrogen, particularly in sectors such as transportation, industry, and power production.
Hydrogen has potential as a multifaceted energy storage medium, effectively mitigating the inherent intermittency of renewable energy sources.
Hydrogen fuel cell cars provide an environmentally friendly mode of transportation that effectively addresses the issue of emissions by producing no harmful byproducts. Furthermore, these vehicles possess the advantageous characteristic of being capable of traveling great distances without the need for frequent refueling.

Technology , Know-How
SNGH2 offers a comprehensive range of top-of-the-line green hydrogen solutions. Our expertise in the fields of electrolyser components design, PEM, SOEC, AEM, fluid dynamics, heat transfer, and physio-chemical reactions as well as internal system design allow us to tailor green hydrogen solutions to your exact needs.
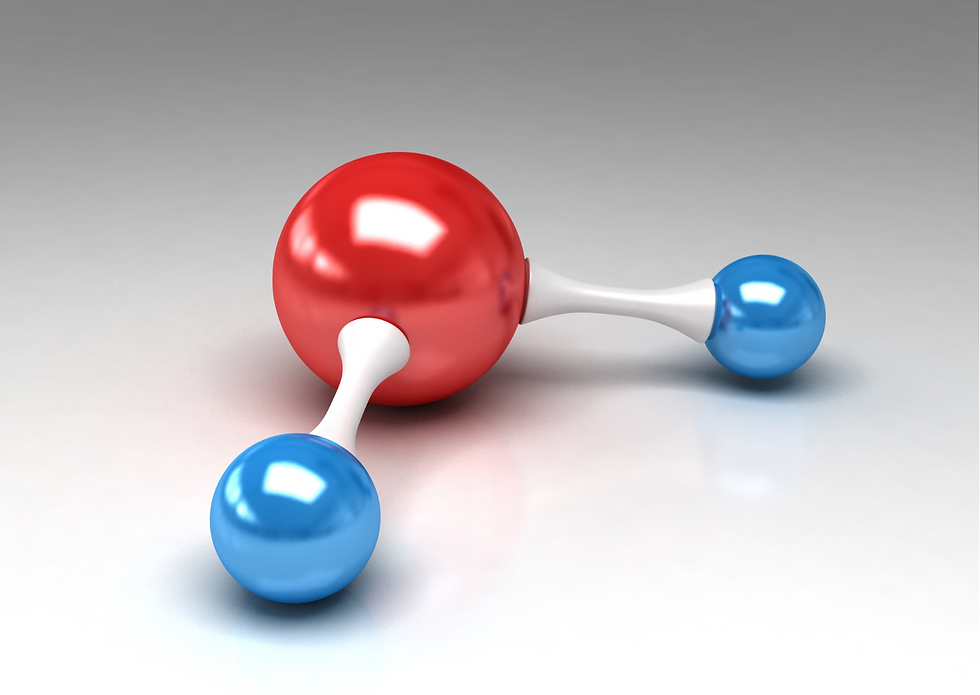
Green Hydrogen Pilot
Pilot projects help understand issues associated with the value chain - operations, technology readiness and gaps, implementation and infrastructure challenges, and integration with the existing systems. Also, the project's commercial viability is understood as part of piloting so that appropriate decisions can be taken for full-scale commercial deployment. The downstream value chain of the green hydrogen ecosystem varies from industry to industry and for different sectors. Our experts provide consulting and solutions for piloting green hydrogen projects in various sectors, such as long-range heavy-duty mobility, steel, energy storage and shipping etc.

Risk Management & Finance
Risk Management plays a vital role in energy security, energy transitions, environmental sustainability and securing and managing project finance for clean energy. Risk management ensures that the path to sustainable energy and ecosystem does not overlook critical risks and parameters associated with the environment, economic growth, and social (including sociopolitical) and legal factors.
The probability of harmful effect on humans or environment resulting from negative externalities is defined as environmental risk. Some of the examples are exposure to radiation, greenhouse gas effects etc. Risk assessment refers to the statistical evaluation of the probability of the harmful event happening. Our expert risk management team integrates the risk assessment with other deliberations such as sociopolitical, economic and legal aspects to make informed decisions on risk identification and reduction, and then making effective communications to the relevant stakeholders.